Robotic-assisted percutaneous coronary intervention (R-PCI) was first successfully performed in 2004, with the first commercially available robotic platform gaining Food and Drug Administration (FDA) approval in 2016.1,2 The shift from a high-radiation exposure bedside procedure requiring manual skills to manipulate intracoronary equipment to a zero-radiation procedure with mechanical robotic control of intracoronary equipment represented a revolutionary change for operators performing percutaneous coronary intervention (PCI).3
The key advantages of R-PCI include a reduction in occupational hazards of radiation exposure and orthopaedic injuries, enhanced mechanical stability, precision and reproducibility for the manipulation of devices, along with the exciting potential for tele-stenting procedures, particularly for underserved geographical regions.3–6
The safety and efficacy of R-PCI has been confirmed in multiple large real-world registries, which have consistently demonstrated high rates of procedural and technical success.7–10 As the number of robotic installations increases worldwide, combined with growing operator experience and technological developments, the range and complexity of lesions and procedures that can be treated robotically is rapidly expanding.9,11–13
In this review, we summarise the latest developments in R-PCI with a focus on developments in robotic technology, procedural complexity, tele-stenting and training methods, which have all contributed to the global expansion in R-PCI (Figure 1).
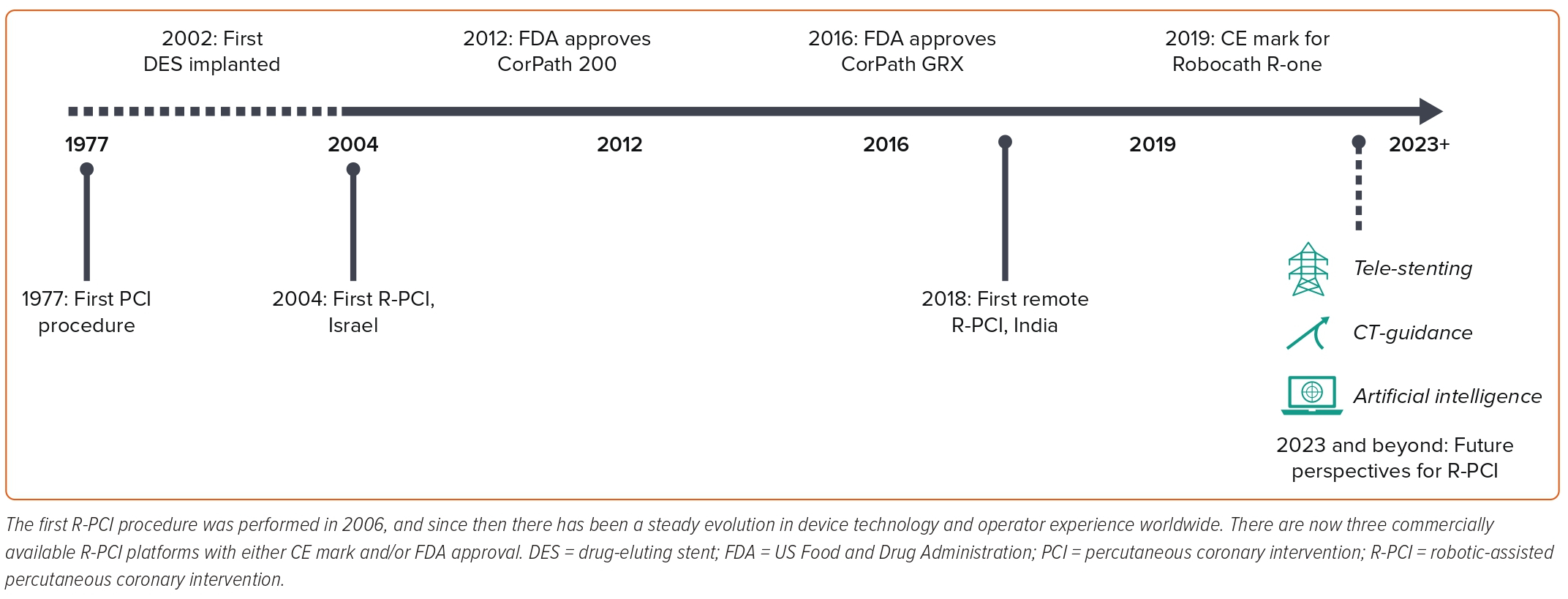
Technological Developments
The first R-PCI procedure was performed in 2004. Since then robotic technology has evolved, with the first commercial robotic platform, CorPath 200 (Corindus Vascular Robotics), receiving FDA approval for PCI in 2012 (Table 1).1,7
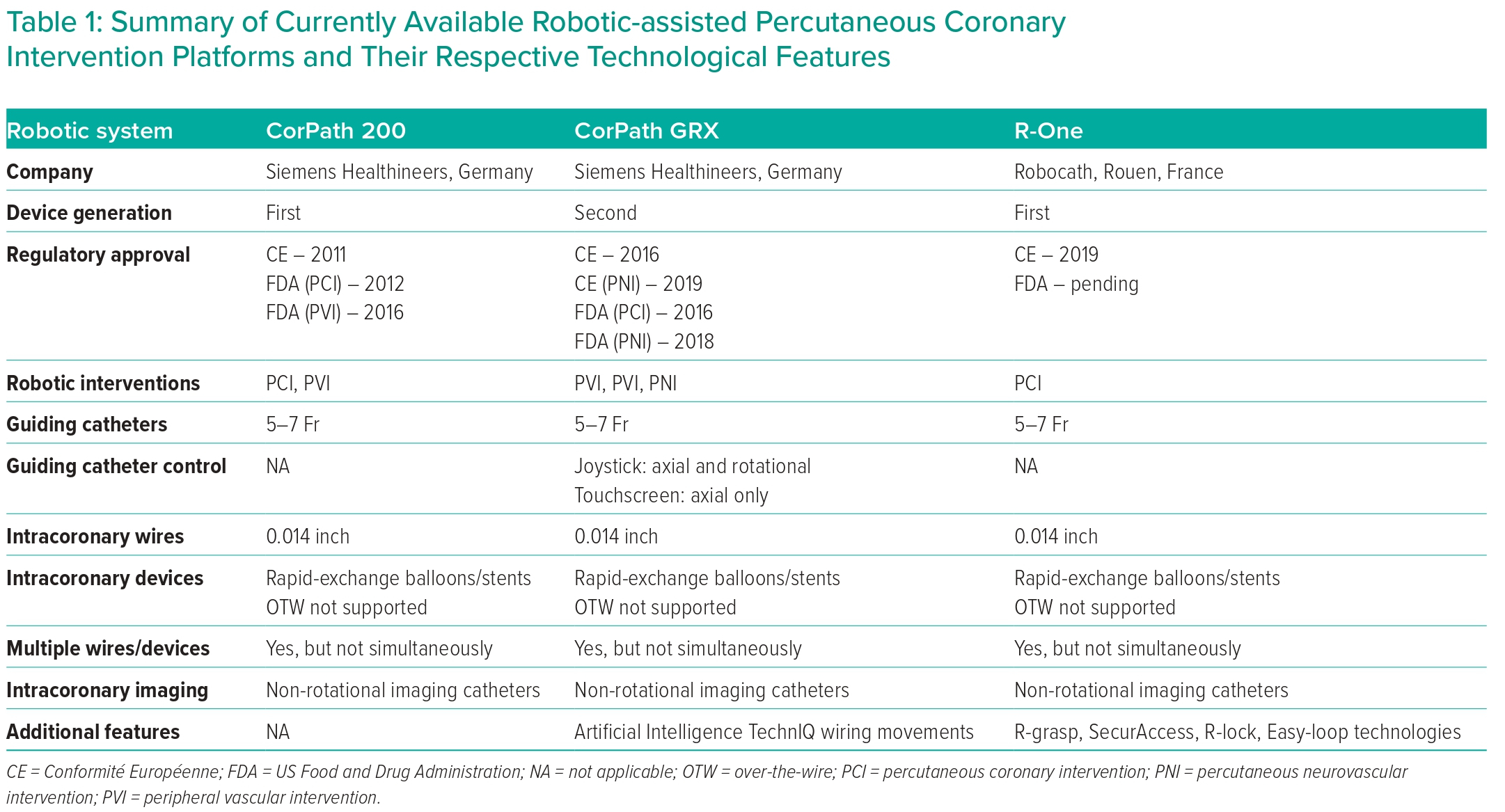
The CorPath 200 consists of two units: a robotic arm, which is positioned at the patient’s bedside, and the robotic cockpit, which can be positioned either inside or outside the cath lab. The robotic arm contains all the intracoronary guidewire and devices, which are then manipulated using the joystick and controls located on the robotic cockpit. The separate location of the robotic arm and robotic cockpit meant that, for the first time, an interventional operator could manipulate intracoronary guidewires and devices without having to be positioned within the radiation field at the patient’s bedside. The R-one (Robocath) device, which received CE mark approval in 2019, also allows operators to control an intracoronary wire and device, but has a more simplified set-up to reduce the learning curve associated with implementing R-PCI in the cath lab.
The newer-generation CorPath GRX R-PCI platform, which received FDA approval in 2016, integrated guiding catheter control, giving operators axial and rotational control of guiding catheters for the first time.12 Further upgrades have also been made to robotic wiring control, with the addition of new automated wiring techniques, which replicate common manoeuvres performed by operators during manual PCI (M-PCI).12 Finally, additional modifications include active device fixation of a wire and the ability to use and control microcatheter systems. To date, these modifications have only been used for percutaneous robotic neurointerventional procedures, but their use in R-PCI is shortly expected.
Despite the aforementioned improvements in robotic technology, there are still significant technical challenges to overcome, which limit the application of robotic platforms for the entire spectrum of coronary and endovascular interventions. Current-generation R-PCI platforms are incompatible with rotational-based imaging catheters, orbital or rotational atherectomy devices and conventional over-the-wire devices. Furthermore, an operator can only actively control a single wire or device at any given time. The additional wires and/or devices stored in the passive drive cannot be manipulated from the robotic console.
Artificial Intelligence and R-PCI
Intracoronary wiring and device manipulations are considered key skills for PCI operators. The transition from manual tactile feedback to robotic visual feedback represents a significant learning curve associated with R-PCI because the technical skills and motor memory often acquired after extensive clinical experience have to be replaced with joystick and touchscreen controls.
Intracoronary wiring with first-generation robotic platforms is conventionally performed by advancing, retracting or rotating a joystick (or by using touchscreen controls), which translates operators’ movements into axial or rotational forces upon an intracoronary wire or device. Although the speed and amplitude of the movements can be controlled, the actual wiring technique or patterns of rotation cannot be altered.
In contrast, with the upgraded CorPath GRX, artificial intelligence technology has been used to combine the conventional axial and rotational joystick movements with different patterns of wiring techniques. Different techniques, termed TechnIQ, have been designed to replicate many of the common movements and manipulations performed by operators. The intracoronary wiring techniques include spin, wiggle and rotate on retract, and can be applied to any wire (0.014" or 0.018") placed within the active wire drive.
In the spin technique, as the operator advances the joystick, the wire rotates 360° clockwise three times, followed by 360° anticlockwise three times. This feature is particularly useful for routine wiring and navigation, particularly through vessels with larger lumens and tortuosity. The wiggle technique involves rapidly alternating clockwise and anticlockwise rotations being applied to the wire as its advanced. These faster, yet lower-amplitude, rotations are particularly helpful when navigating through a tight lesion or stenosis inside smaller vessels. Rotate on retract works when an intracoronary wire is being pulled back and an automatic 270° rotation is applied to change the direction of the tip of the wire. This technique is particularly effective when wiring a vessel and multiple side branches are encountered. If the wire enters into a side branch, the rotate on retract feature will automatically rotate the wire as the operator pulls the wire out of the side branch to redirect the wiring trajectory towards the distal segment of the vessel.
Similarly, robotic device control is limited to axial movements only, and only the speed of device movement can be altered in a linear manner depending on how much pressure is applied to the device joystick. Two further TechnIQ techniques have been developed to enhance device control, namely dotter and constant speed, and both can be applied to any rapid-exchange device (balloon, stent or intracoronary imaging catheter) placed within the active device drive.
The dotter technique applies a rapid alternating ‘back-and-forth’ movement to the intracoronary device as it is advanced and replicates a common technique used by operators to facilitate crossing of difficult lesions, tight stenosis, calcific segments or stent struts.
Constant speed applies a continuous and prespecified velocity (2 or 5 mm/s) of movement to any device when the joystick is advanced or retracted. This is particularly useful when performing intravascular imaging assessments, whereby a set pullback velocity can be applied to the imaging catheter for more detailed and accurate measurements to be performed.
The different wiring and device TechnIQ movements can be simultaneously combined with the joystick and touchscreen controls; in addition, the operator can easily and instantly switch between different TechnIQ manipulations in order to select the most appropriate wiring and device strategy to tackle the intracoronary challenge (Figure 2).12
The safety and procedural effectiveness of these TechnIQ movements were evaluated in the NAVIGATE-GRX randomised clinical trial (NCT04883008). Fifty patients undergoing R-PCI with the CorPath GRX were randomised to TechnIQ on versus TechnIQ off. Procedural safety, clinical success and technical success were equivalent for both groups. The use of TechnIQ was associated with a 26% reduction in procedural time, a 31% reduction in fluoroscopy time and a 21% reduction in radiation exposure.14 Furthermore, the requirement for manual input, defined as the need to complete a particular step of the PCI procedure manually, was less frequently observed in the TechnIQ on group. Further iterations of TechnIQ and deeper integration of artificial intelligence technologies are expected to further increase the technical capabilities of R-PCI.
Complex PCI Cases
The aforementioned technological developments, combined with the growing operator experience worldwide, have fuelled an increase in the range and complexity of lesions being treated robotically (Table 2).9 Treating complex lesions often requires prolonged procedural times, higher radiation doses, increased use of devices, the need for precise measurements with accurate and stable device deployments, all of which can benefit from robotic technology.
During R-PCI, the operator is comfortably seated at the robotic cockpit in front of a large screen displaying the angiographic images in combination with the haemodynamic and intracoronary imaging or physiology data in real time. The benefits of this enhanced visualisation, operator comfort and working ergonomics are particularly noticeable when performing complex and/or multivessel PCI procedures, which often require more lesion preparation, the use of adjunctive equipment, longer stents and higher rates of post-dilatation.
Robotic sub-millimetre precision combined with touchscreen controls allows for accurate lesion measurement and device positioning, which can be useful when performing ostial or complex two-stent PCI procedures. Replacing visual angiographic-based lesion measurements with robotic mechanically based measurements can potentially eliminate the errors associated with lesion foreshortening. In a propensity-matched analysis comparing M-PCI to R-PCI performed using a first-generation robotic platform, R-PCI was associated with a significant reduction in the incidence of longitudinal geographical mismatch.15
In addition, current-generation R-PCI platforms are compatible with the entire range of 0.014" intracoronary wires, rapid-exchange devices, guide extension catheters, intracoronary physiology wires and specific non-rotational-based intravascular imaging catheters. Finally, the ability to store multiple additional wires and/or devices in the passive drives of the R-PCI platforms allows operators to perform more complex procedures, such as bifurcation PCI.13
These advantages have resulted in R-PCI being used to treat an increasing number of complex coronary lesions, including multivessel disease, bifurcations, heavily calcified, un-protected left main stem, acute ST-elevation, chronic totally occluded and ostial lesions (Figure 3).8,9,11–13,16–18
Studies have consistently demonstrated high rates of safety and technical and procedural success for R-PCI of complex coronary lesions. In the CORA-PCI study, 157 lesions, of which 123 (78.3%) were type B2/C complexity, were treated robotically with a clinical success rate of 99.1%.9 The technical success rate (defined as procedure completed robotically or with partial manual assistance in the absence of major adverse cardiovascular events) was 91%, and the two main reasons for requiring manual input were inadequate guiding catheter support and requirement for simultaneous manipulation of multiple devices. Of note, the first issue has been addressed with the second-generation CorPath GRX, which allows for guiding catheter control, whereas the latter remains an issue for further iterations of R-PCI platforms. When R-PCI was compared to M-PCI for complex lesions, again safety and clinical success rates were similar but R-PCI was associated with reduced contrast volume and radiation exposure but prolonged procedural times. However, following propensity-matched analysis for the most complex of lesions, no significant difference remained between R-PCI and M-PCI.10
An emerging concept for treating the most complex of lesions, such as calcific left main bifurcation disease, is that of a hybrid robotic and manual approach.13 In these complex scenarios, an operator can prepare a procedural strategy, outlining which steps will be performed robotically and which steps have to be performed manually. Execution of the procedural strategy requires real-time two-way communication between the robotic cockpit operator and bedside operator, who is responsible for the loading and exchanging of wires and devices within the robotic cassette. This hybrid approach allows an operator to combine the strengths of a robotic platform, such as precision and stability, and to overcome some of the limitations of current-generation R-PCI platforms, such as the inability to use rotational imaging catheters, over-the-wire devices, atherectomy devices or simultaneous control of multiple wires and/or devices.
CT-guided R-PCI
CT-guided revascularisation is gradually gaining ground in the field of coronary interventions.
The combination of CT-based 3D reconstruction and CT-based computational fractional flow reserve (FFR-CT), assessing anatomy and physiology in one study, can play an important role in both risk stratification and procedural planning. Having a coronary CT angiogram (CCTA) before the patient even enters the cath lab opens the possibility to characterise the plaque in a way comparable to intravascular imaging. Metrics, such as plaque morphology with imaging of existing calcification, lesion length, minimum lumen diameter (MLD) and reference vessel diameter (RVD), are readily available and can be used to guide the procedure with the aim of maximising precision and predictability. Based on this information, a preprocedural CCTA-based strategy could be formulated that would influence the choice of materials and the overall patient journey (selecting those patients who can be treated in a same-day setting), with a resulting impact on costs. In addition, a novel approach for CT-guided revascularisation is the synchronisation of a CCTA-derived 3D reconstruction with the angiographic C-arm to facilitate lesion crossing and stenting.19,20
The combination of detailed CT planning with the precision of R-PCI represents a fundamental breakthrough in the diagnostic and therapeutic pathway for patients with coronary artery disease (Figure 4). From an operator’s perspective, the presence, distribution and detailed anatomical plaque characteristics can be obtained without stepping foot inside the cath lab and without incurring any radiation exposure. The entire procedural strategy from approach to choice of materials, including guiding catheters, wires, balloon and stent sizes and lengths, to calcium modification can all be planned upfront, and the entire procedure executed from the 5 cockpit, which can be positioned outside the cath lab, again incurring zero radiation exposure. In addition, the combination of CT-derived angulations and the synchronisation of the C-arm with the 3D reconstruction derived from CT may lead to further reductions in contrast volume and procedural time during R-PCI, an effect that may be further magnified when treating more complex lesions.21 The sub-millimetre precision of CT data can be used to accurately plan stent sizes and landing zones, which can then be easily executed precisely and reliably using the robotic mechanical controls, especially the 1 mm touchscreen movements.
The potential impact of combining CT with R-PCI on procedural outcomes, clinical safety and success remains to be determined and further studies are awaited. In the ongoing P4 study (NCT05253677), which is evaluating the non-inferiority of using a CT-guided approach compared to intravascular ultrasound-guided PCI on procedural outcomes, the use of R-PCI is left to the discretion of the operator and procedural and clinical outcomes will be evaluated. Finally, the biggest rationale for combining CT with R-PCI may be for remote or tele-stenting procedures, where CT may eventually replace the diagnostic angiogram for the remotely connected robotic operator.
Tele-stenting
Even within developed countries, access to acute endovascular therapies for MI and stroke remains limited in rural areas.22 Consequently, patients in rural locations may not be offered catheter-based treatments or may require transfer to receive them, which can be problematic because delays in reperfusion result in higher mortality and greater infarct size in MI and greater mortality and disability in stroke.23,24
One proposed method to improve access to endovascular procedures in rural geographies is through tele-robotics, wherein an endovascular intervention is performed using a combination of robotics and telecommunications by a physician operator in a separate geographic location from the patient.
The possibility of performing tele-robotic PCI (i.e. ‘tele-stenting’) was first investigated in a small pilot study in which the interventional cardiologist and robotic controls were removed from the procedure room housing the patient and placed behind the closed doors of an isolated separate room.6,25 In this configuration, the operating physician was located approximately 17 m from the patient, and all communication between the physician and procedural staff took place via telecommunications devices. This small study demonstrated, for the first time, that it was feasible for a physician to perform robotic PCI from outside the confines of the procedure room and that current technology no longer required the operating physician and patient to be in the same location for PCI to occur. A significant limitation of this study was the physical connection imposed by the cables connecting the robotic controls to the robotic arm.26
Further adaption of the robotic system subsequently enabled communication of the robotic controls and robotic arm over a network, thereby eliminating a direct cable connection of the robotic components. In May and June of 2018, the feasibility of long-distance tele-stenting over a network was demonstrated in experimental models, including the successful performance of tele-robotic PCI in an in vivo swine model over a distance of 165 km.25 in December 2018, tele-robotic PCI in five humans was then successfully demonstrated over a distance of approximately 30 km.27 Now that the feasibility of tele-robotic PCI has been demonstrated, additional studies of tele-robotic PCI in humans are needed.
Contemporary networks have become fast enough to enable tele-robotic capabilities over distances of >4,800 km, as recently demonstrated in an ex vivo experimental model in which the robotic operator and controls were in Boston and the robotic arm and simulated patient were in San Francisco.28 Regardless of whether performed over contemporary land-based networks or over 5G wireless networks, round-trip latencies of <200 ms were demonstrated in that study, well below the latency values previously shown to be perceptible by robotic operators.28–30 These findings suggest that the geographic reach of tele-robotic endovascular interventions could be profound. Emerging research has suggested tele-robotic interventions may not be limited to PCI, because preliminary studies of tele-robotic endovascular interventions for the treatment of stroke and peripheral arteries disease have now been conducted.31,32 Further development of tele-robotic capabilities may eventually facilitate the dissemination of interventional expertise and improve access to endovascular procedures worldwide.
Simulation Training for R-PCI
The differences in equipment, techniques and cath lab workflow and dynamics between R-PCI and M-PCI constitute the learning curve associated with R-PCI. An operator has to adjust from directly controlling intracoronary wires, devices and catheters to interacting with a robotic console and arm. Therefore, new methods of training and education are required to help operators, and the wider cath lab team, acquire the necessary skillsets required for R-PCI.
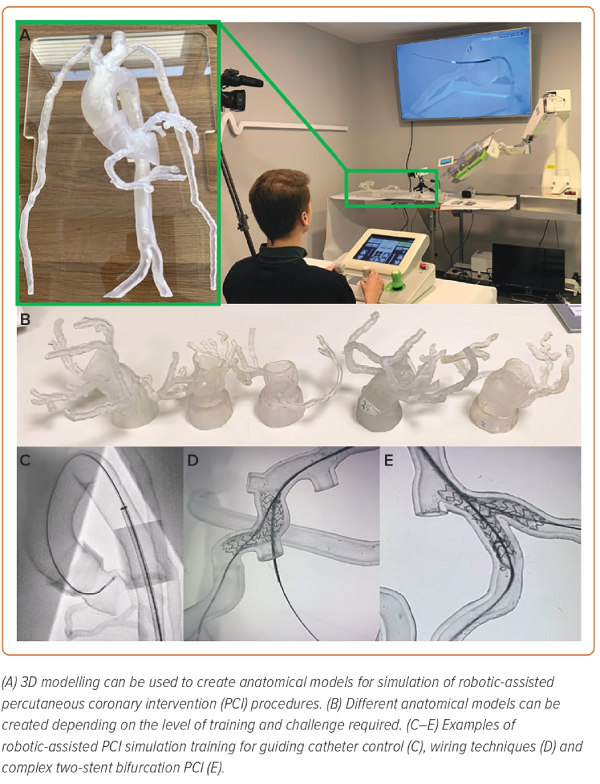
Simulation training with the use of silicone-moulded or 3D-printed anatomical models is increasingly being used for training and education in interventional cardiology (Figure 5).33–36 3D modelling is more realistic and, with improvements in material technology, is closer to the in vivo feel compared with digital or electronic simulators.34,37 Furthermore, 3D modelling requires fewer resources and has fewer ethical concerns than preclinical animal models, with the key advantage being the ability to perform the same procedure repeatedly within the same model, which can accelerate an operator’s progression along the learning curve. 3D models are highly flexible and customisable, allowing for different anatomical scenarios or situations to be replicated depending on the training needs (Figure 5A,B).36 Coronary phantoms that closely replicate the dimensions, trajectory and in vivo feel of coronary arteries can be developed based on real patient coronary CT scans to practice different robotic wiring techniques. Complex wiring scenarios, such as recrossing stent struts or navigating through highly tortuous vessels, can also be simulated (Figure 5C–E). The ability to perform multiple wiring procedures in the same models also allows operators to learn the benefits and drawbacks of the different robotic wiring controls, as well as to better understand which technique to use in certain anatomical scenarios.
Models can also be created with different aortic sinus and coronary ostial anatomies to allow operators to perform multiple repeated guiding catheter cannulations using different sizes and shapes of guiding catheters in each of the models.38 This experience allows operators to familiarise themselves with the mechanical forces involved in robotically controlling a guiding catheter, which is particularly useful when approaching more complex lesions. As an operator transitions from a basic user to a more advanced robotic operator, more complex anatomical scenarios and lesions can be developed, such as two-stent bifurcation PCI, to further develop their skillset and ease with the robotic system.
These 3D models can now be integrated with high-fidelity simulators to allow for training to take place in a cath lab environment complete with fluoroscopy and interventional equipment, including a robotic platform. This high-fidelity training allows not only operators, but also the wider cath lab team to train and develop the necessary skills required when transitioning to an R-PCI programme, which completely changes the dynamics within the cath lab. New working practices and standard operating procedures can be practised, rehearsed and subsequently implemented following simulation training. In many institutions, the role of the bedside operator managing wire and device exchanges is undertaken by nursing staff, which requires additional training to be undertaken in the basic equipment and techniques used for PCI. In this regard, simulation training can also play an important role in building confidence with equipment and procedures, to ensure a smooth transition for the cath lab team from M-PCI to R-PCI. In addition, complications and emergency scenarios can be recreated to rehearse team dynamics and determine the optimal workflow, something that cannot be replicated in humans or easily with animal testing.
Given that R-PCI is still in its early stages, new technological hardware and software developments are expected and operators are likely to discover new techniques during R-PCI.38 3D modelling with simulation provides the ideal platform for education, the training of robotic operators, particularly for complex PCI, and to safely explore and potentially speed up further technological development of R-PCI.