Coronary angiography has been the gold standard technique for evaluating coronary arterial disease for the past 50 years. Increasingly, however, realisation of the limitations of coronary angiography, mainly the inability to supply information regarding the coronary wall, has prompted the design and development of adjunctive technologies to better evaluate not just luminal disease but also the burden and character of atherosclerotic plaque within the vessel. The development of intracoronary imaging modalities, namely intravascular ultrasound (IVUS) and optical coherence tomography (OCT), has progressed quickly and these technologies now have established roles in the diagnosis and treatment of coronary artery disease. In general, intracoronary devices that can assess the coronary endothelium use either acoustic or optical signals that are received by a coronary catheter (IVUS uses ultrasound, OCT uses near-infrared light). This review addresses these two widely used intracoronary imaging techniques, looking at their clinical applications, recent evidence for their use and describes new developments in the field.
Intravascular Ultrasound
In the last 25 years IVUS has been established as the most commonly used intracoronary imaging device. An IVUS system consists of a flexible monorail catheter with an ultrasound transducer at its tip that emits ultrasound waves in the 10–40 MHz range and an electronics console to reconstruct the image (see Figure 1).1 After reflection from tissue, part of the ultrasound energy returns to the transducer and is converted into the image.
There are two types of IVUS transducers for clinical use: the mechanical rotating transducer and the electronically switched phased array system. The mechanical transducer uses a single crystal on a rotational device, which visualises the entire vessel in cross-section providing better image quality (compared with phased array technology) of 100–150 μm.2 The main disadvantage of mechanical transducers is the central drive shaft that decreases flexibility and prevents the concurrent use of a central guidewire.3 However, newer rotational IVUS catheters have developed a monorail system that allows for the presence of a central guidewire. Phased array catheters use multiple transducer elements, which are mounted along the circumference of the catheter tip. Each element sends and receives ultrasounds from a sector and multiple sectors are gathered to produce a cross-sectional image of the artery. However, they are disadvantaged by a technically complex set-up, requiring detailed programming;3 but some of the newer catheters are easier to set up.
Intracoronary imaging of coronary vessels by IVUS is performed using standard coronary interventional techniques and equipment (guiding catheter and 0.014 inch angioplasty guidewire) for catheter delivery along the guidewire beyond the target lesion/area of interest. Intravenous heparin and glyceryl trinitrate (nitroglycerin) are routinely administered before imaging. The IVUS catheter is then drawn back across the target lesion by either an automated pullback device (usually at a rate of 0.5–1.0 mm/s for any length) or by manual operator pull back. Importantly, as ultrasound waves pass through water and blood without major rebound signal, no coronary preparation is needed during image acquisition.
Safety of Intravascular Ultrasound
There is good evidence for the safety of IVUS use.4 Major complication rates (such as coronary artery dissection) are reported as <0.5 %.3 Minor complication rates vary from 1 to 3 % and are mainly due to coronary artery spasm, which is generally transient and responsive to intracoronary administration of nitrate.
Uses of Intravascular Ultrasound
Characterisation of Atherosclerosis
IVUS can be used to measure plaque extent, morphology and distribution,5–7 and importantly provides information about plaque composition. This is because denser material such as calcium reflect more ultrasound waves, which results in a higher intensity image. Additionally, calcium does not allow any ultrasound waves to penetrate to deeper tissue, hence producing an acoustic shadow. On the other hand, lipid-laden lesions appear hypoechoic and fibromuscular lesions generate low-intensity or ‘soft’ echoes.3 Lipid-laden or fibromuscular lesions may exhibit a prominent echogenic fibrous cap, although most fibrous caps are too thin to be resolved by IVUS. IVUS therefore allows important decisions to be made with regard to intervention – for example, if calcium is identified, then rotational atherectomy could be considered.3
Apart from the greyscale image used for plaque interpretation, extensive research investigating ways of improving the assessment of plaque composition by IVUS has been performed. The Kawasaki group at the Gifu University Graduate School of Medicine in Japan has published studies using integrated backscatter signals from the radiofrequency signal of ultrasound, and based on the backscatter IVUS image, they have used colour to code different components of plaque.8,9 Another established technique developed by Volcano Corporation® (Rancho Cordova, CA, US) uses radiofrequency signals to determine plaque composition.10 In this technique, the distortion of radiofrequency signal by the plaque is passed through an algorithm, which is then colour-coded and superimposed on the grey image – a technique commercialised as ‘Virtual Histology intravascular ultrasound’ (VH-IVUS).10 Recent imaging technology now allows the reconstruction of VH-IVUS images in a longitudinal view, enabling a more comprehensive analysis of the total length of the plaque, its spatial orientation and its relation to the rest of the coronary artery. The potential of this imaging modality for analysing plaque vulnerability was demonstrated in a recent study where VH-IVUS backscatter data from ex vivo left anterior descending coronary arteries were recorded and compared with histological interpretation of the same sites.11 The overall predictive accuracies for VH-IVUS were 93.5 % for fibrotic tissue, 94.1 % for fibro-fatty tissue, 95.8 % for necrotic core and 96.7 % for dense calcium.11 Further data were provided by the Carotid Artery Plaque Virtual Histology Evaluation (CAPITAL) study,12 where a strong correlation between VH-IVUS plaque characterisation and characterisation following direct histological examination of the plaque (following endarterectomy) was demonstrated with a predictive accuracy of 99.4 % for thin-cap fibroatheroma (TCFA), which is thought to be the precursor lesion of plaque rupture, and 96.1 % for calcified TCFA.12
Vessel Dimensions
Although angiography allows measurement of luminal diameters in two-dimensional views, IVUS produces a tomographic view, which provides higher resolution as well as precise vessel and plaque dimensions.13 Therefore, the true minimal and maximal luminal diameter can be measured with IVUS. Furthermore, the cross-sectional area measurement of the lumen as well as the vessel can be obtained.13
In addition, IVUS has been useful in demonstrating diffuse disease in angiographically, ‘normal’ arteries, which may have as much as one-third of their cross-sectional area filled with diffuse plaque.14,15
Does Intravascular Ultrasound Use Improve Outcomes?
Identifying Vulnerable Plaque
The Providing Regional Observations to Study Predictors of Events in the Coronary Tree (PROSPECT) trial, used angiography, three-vessel greyscale and radiofrequency IVUS to evaluate the natural history of atherosclerosis in a prospective group of 697 patients with acute coronary syndromes who underwent percutaneous coronary intervention (PCI) and subsequent optimal medical therapy. During a median follow-up of 3.4 years, culprit lesions at the time of initial study were felt to be related to major adverse cardiac events (MACE) in 12.9 % of patients, with non-culprit lesions responsible in 11.6 %. Aftermultivariate analysis, non-culprit lesions associated with recurrent events were more likely to have three characteristics: a minimal luminal area of <4 mm2; a plaque burden of >70 %; or classified as TCFA. Furthermore, those lesions that were responsible for future MACE were observed to be mild when assessed by angiography (mean diameter of stenosis 32 ± 21 %), but using IVUS, these lesions had a plaque burden of 67 ± 10 %. At the time of follow-up, these lesions had progressed angiographically to a mean angiographic diameter stenosis of 65 ± 16 %.16 It is important to note, however, that while IVUS has been observed to be a validated tool to predict lesions responsible for future MACE, it is not able to image well through calcium, nor is it accurate in identifying thrombus.17
Assessment After Percutaneous Coronary Intervention
In a randomised trial studying drug-eluting stent (DES) deployments with or without IVUS guidance in 210 patients, IVUS use led to more frequent post-dilations, higher balloon inflation pressures and the use of larger balloon sizes. However, despite this there was no significant difference in MACE rates (11 versus 12 %; p = not significant) at 18-month follow-up.18 A further retrospective study found no significant differences in the rates of restenosis with and without optimal stent expansion guided by IVUS in 250 patients undergoing PCI with DES.19
Although currently insufficient evidence exists to support a reduction in the rates of restenosis with IVUS use there is some evidence supporting IVUS guidance to reduce rates of stent thrombosis. In one study of 884 patients with DES implantation, IVUS-guidance was associated with less direct stenting, more post-dilation, greater cutting balloon and rotational atherectomy use. At 30 days and 12 months, lower rates of definite stent thrombosis were seen in the IVUS group (0.5 versus 1.4 %; p=0.046 and 0.7 versus 2.0 %; p=0.014, respectively).20
Optical Coherence Tomography – a New Era of Intracoronary Imaging
OCT was first developed by two Japanese researchers at the Yamagata University (Japan) and subsequently at the Massachusetts Institute of Technology in the US in 1991. In vitro OCT was initially performed in the retina but adopted in the coronary artery later in the same year.1,21 Instead of ultrasound (like IVUS), OCT uses near-infrared light, which is absorbed by water, lipids and erythrocytes (see Figure 2). The high-resolution of OCT has allowed use of this technology for both clinical and research purposes.21 OCT has widely been used in the assessment of coronary anatomy over the last decade, and has a wide range of clinical applications including coronary plaque anatomy, post-PCI stent position and malapposition. Within research, OCT has been able to improve the evaluation of stent endothelisation post-implantation. Although initial OCT systems consisted of time-domain optical coherence tomography (TD-OCT) technology, this has been surpassed by frequency-domain optical coherence tomography (FD-OCT) technology.
Current OCT catheters are 3.2 Fr flexible short monorail systems with an optical emitting transducer that emits a near-infrared wavelength of about 1,300 nm. Unlike the IVUS catheter, the OCT catheter transducer lies 20 mm behind the distal marker. The transducer contains optical fibres with a micro-lens transducer that is placed beyond the target lesion along a standard guidewire. The OCT catheter does not move during image acquisition, instead the transducer moves back inside the central part of the catheter. The catheters have an automated pullback system at a rate of 25 mm per second with an image range of 50–70 mm when adequate coronary preparation has occurred. As the light source is easily absorbed by blood there is a need for coronary preparation prior to image acquisition. The use of pure contrast through a manifold to prepare the coronary artery with total blood removal is generally recommended with most left coronary systems requiring 10–14 mls and right coronary arteries 8–10 mls. The OCT system consists of an OCT imaging catheter (ImageWite TM, St Jude TM, St Paul, Minnesota, US) and an OCT system console, which contains the optical imaging engine and computer signal acquisition (M2/M3 CV OCT Imaging System, LightLab Imaging, Inc, Westford, Massachusetts, US).
Image Acquisition Limitations of Optical Coherence Tomography
Due to the need for complete coronary preparation, if any blood pooling remains, a high signal will remain within the image distorting the final image. In addition, as the guidewire does not run through the entire length of the OCT catheter, all images will have a silhouette of the guidewire with reduction of image quality in these areas (see Figure 3).
Safety of Optical Coherence Tomography
The relatively low energy used in OCT (5.0–8.0 mW) does not cause functional or structural damage to the coronary tissue. The main safety concern with OCT is the use of a contrast bolus in coronary preparation – however, studies have shown that no patients suffered contrast-induced nephropathy, but there is a relatively small risk of coronary spasm and electrocardiogram (ECG) changes during contrast administration.22
Assessment of Coronary Lesions with Optical Coherence Tomography
Plaque Characterisation
Since there is greater spatial resolution with OCT compared with IVUS (see Figure 3), OCT can provide more detail regarding the microstructure of the vessel wall and specifically OCT has been shown to identify TCFA, a feature not possible by IVUS. Studies have shown a high degree of correlation between OCT imaging and fibrous cap thickness on histologic evaluation.1,17,23 In addition, OCT can identify TCFA by measuring the thickness of the fibrous cap and the arc of the lipid-rich plaque.24–26 Lipid pools are less sharply delineated than calcification and show lower signal intensity. Lipids also exhibit more heterogeneous backscattering than fibrous plaques.27,28
OCT has been shown to be helpful in determining prognosis by identifying vulnerable plaques. A prospective study of the characteristics of non-culprit lesions in 53 patients with coronary artery disease undergoing PCI showed that TCFA (as assessed by OCT) and the presence of micro-channels had a significant correlation with plaque progression (defined as >0.4 mm increase in minimal luminal diameter) at a seven-month follow-up.29
Thrombus
Thrombus is well visualised by OCT with the technique able to distinguish between different thrombus phenotypes.25,26,30 OCT images for white thrombi (composed of platelets and leucocytes) produce a signal-rich mass whereas red thrombi (containing mainly erythrocytes) produce high backscattering protrusions with strong signal attenuation.31 If there is a large red thrombus, then this may interrupt the visualisation of the characteristics of an underlying plaque due to signal attenuation. It is possible to misinterpret mural thrombi as lipid-rich fibroatheroma, due to similar OCT single attenuation patterns produced by these two plaque components. Therefore, thorough examination of the structures and surface are required to differentiate between these two pathologies.
Vessel Sizing
OCT allows clear delineation between the lumen and vessel wall, although due to shallow penetration there may be a limit in the detail of the whole vessel structure visualised as compared with IVUS imaging.32 OCT also provides accurate measurement of reference lumen diameters with studies showing that for proximal culprit lesions, TD-OCT measurements were almost identical to those measured with IVUS.33
Optimising Percutaneous Coronary Intervention
OCT allows detailed evaluation of strut apposition to the vessel wall and stent expansion after stent deployment. As the infrared light cannot penetrate into the metal struts of the stent, the luminal surface shows a strong reflection with shadowing behind the struts and consequently improves the visibility of individual stent struts. After stent deployment, OCT allows visualisation of stent edge dissection, tissue protrusion and incomplete stent apposition that may not be detected by either IVUS or angiography alone.33,34 OCT has also been used as one of the primary imaging modalities for follow-up evaluation of several bioabsorbable vascular scaffolds (BVS), which are being studied in clinical trials. A recently published study35 evaluated 100 lesions from 73 patients comparing BVS with an equal number of matched lesions treated with second-generation DES. OCT of these lesions showed a significantly higher rate of tissue prolapse and higher rates of incomplete strut apposition at the proximal edge in the BVS group. However, there was no difference inthe overall rates of incomplete strut apposition. Therefore using OCT, this study demonstrated that BVS had similar post-procedure area stenosis and minimal lumen area as second-generation DES. Another study, the ABSORB study,36 investigated 30 patients with a single de novo coronary artery lesion treated with BVS, who were followed up for two years clinically and with multiple imaging methods including OCT. At two years after implantation, 34.5 % of strut locations had no discernible features detected by OCT, suggesting a significant reduction in restenosis as well as reducing the risk of late thrombosis.
Stent area measured by OCT could potentially be an alternative endpoint of PCI. This is because OCT has helped to predict no reflow26 post-PCI, based on the presence of TCFA. The clinical significance of these OCT findings and whether they warrant further intervention remains unclear;with a small natural history study showing that these findings resolved without significant restenosis or thrombus formation at six-monthfollow-up.37 To date, no studies have been completed investigating the role of OCT in optimising PCI for non-ST-segment elevation acute coronary syndromes (NSTEACS). The Does Optical Coherence Tomography Optimise Results of Stenting (DOCTORS) study will randomise 250 patients to have OCT-guided angioplasty or angioplasty alone. In addition to the safety of OCT in angioplasty for NSTEACS, the study will also investigate whether OCT yields useful additional information beyond that obtained by angiography alone and whether this information changes interventional strategy.38
Finally, a recent study investigated the use of OCT to guide the management of patients with ACS and large thrombus burden.39 The study involved 852 patients with ACS. Of these patients, 101 had large thrombus burden and underwent thrombectomy to restore Thrombolysis In Myocardial Infarction (TIMI) 3 flow. These patients subsequently had OCT on days 0–2 (acute), days 3–6 (early) and days 7–30 (late). The study found that the delayed group had reduced thrombus burden, resulting in 38 % of patients not requiring stent implantation. This suggests that OCT identified culprit lesion morphology not discerned by angiography alone and therefore OCT facilitated PCI decision-making.
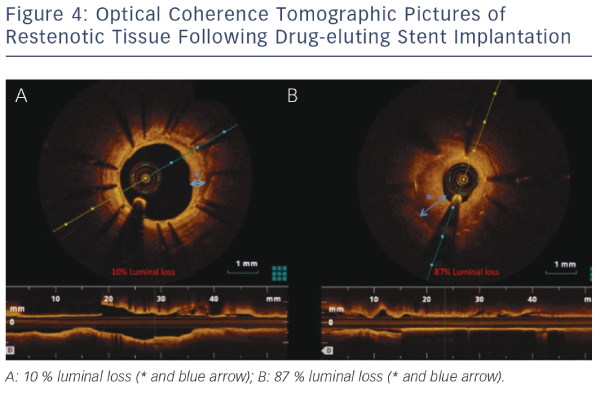
Assessment of Neointimal Coverage with Optical Coherence Tomography
Strut coverage is an important surrogate risk factor of stent thrombosis. According to IVUS examinations, most DES appear uncovered by neointima; however, the limited resolution of IVUS makes it difficult to calculate the thickness or even extent of neointimal coverage. Using OCT, strut coverage is clearly seen and both the coverage of individual struts and the thickness of neointimal coverage can be assessed accurately.40 In one study, at six-months follow-up, 89 % of sirolimus-eluting stents (SES) lesions were covered by thin neointima, and 64 % of the stent struts were covered with neointima that had a thickness of less than 100 μm (which would be undetectable by IVUS).40
Even though the introduction of DES has led to reduced rates of restenosis, this complication following PCI still occurs and our understanding of its pathophysiology is still poor. OCT has helped advance our understanding, with studies demonstrating that stent restenosis is not homogenous. Furthermore, OCT imaging allows separation of restenotic tissue into homogenous, layered and heterogeneous groups. This was demonstrated in a study where paclitaxel-eluting stent restenosis could be easily classified into these three groups using OCT.41Figure 4 demonstrates the sensitivity of OCT in characterising the extent of restenosis after DES implantation.
OCT has been increasingly used as an endpoint in clinical trials of newer generation DES, e.g. the Limus Eluted from A Durable versus Erodable Stent coating (LEADERS) randomised trial comparing a biolimus-eluting stent (BES) with SES. Here, 56 consecutive patients underwent OCT during angiographic follow-up at nine months. At an average follow-up of nine months, strut coverage was more complete in patients allocated to BES compared with those with SES.42 However, whether uncovered stent struts visualised by OCT directly relate to late stent thrombosis after PCI remains largely unclear.
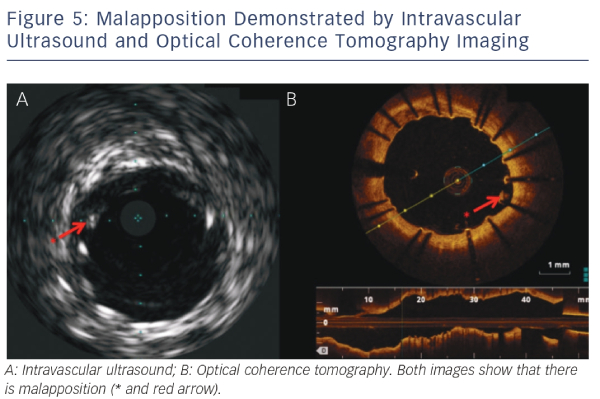
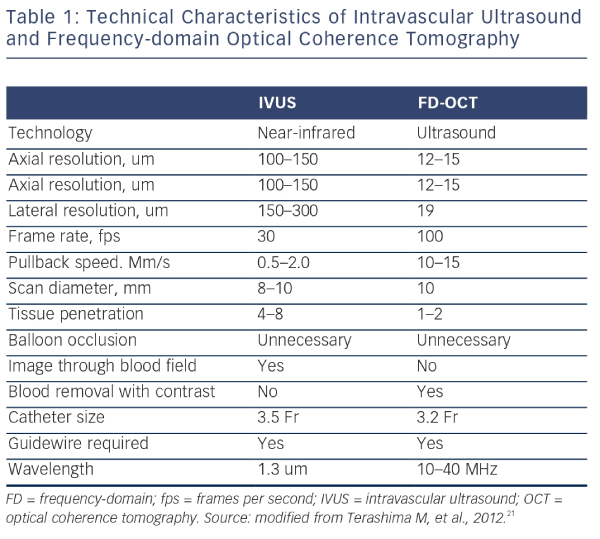
Optical Coherence Tomography Observations of Very Late Stent Thrombosis After Drug-eluting Stent Implant
It is believed that very late stent thrombosis may be due to delayed arterial healing as well as incomplete endothelialisation following stent implantation.43 OCT has been used to observe very late stent thrombosis 29 months after SES implantation. Here OCT showed multiple inter-strut ulcer-like appearances and late strut malappositions.44 These changes could represent OCT signs of very late stent thrombosis.
Although these observations are important to understand differences in stent design, further studies are required to determine the clinical significance of these findings, and in particular, whether information obtained using OCT can be predictive in identifying patients at risk of stent thrombosis or restenosis. Large-scale, prospective studies are needed to address clinical questions such as the relationship between clinical outcome and DES deployment, vascular healing, the time course of endothelial stent coverage, as well as the threshold for stent coverage and late-stent thrombosis.
Comparison of the Two Techniques
IVUS provides useful information regarding vessel size, plaque morphology/area and can be used to guide the selection of interventional strategies; however, it is limited by image resolution. This is where OCT has demonstrated superiority with improved image resolution and contrast, and is therefore more attractive for the assessment of coronary arteries in further detail. The resolution of OCT (10–20 μm) is 10-fold higher than that of IVUS (100–150 μm); however, as a consequence, the penetration depth is lower (OCT: 1–2 mm compared with IVUS: 4–8 mm).25 Therefore, there is a limit in the ability of IVUS to detect intimal tears, thrombus and stent malapposition (see Figure 5) whereas OCT has been demonstrated to visualise intimal hyperplasia, intraluminal thrombi, stent edge dissection and mural thrombus after PCI.22,45,46 Specific differences between OCT and IVUS are shown in Table 1.

With respect to plaque characterisation, OCT allows greater in-depth visualisation of detailed coronary struts including characteristics of coronary plaque (i.e. lipid-rich, fibrous and calcified plaques).25,26 However, in several applications, the shallower penetration of OCT may be a drawback. Whole vessel structures, including the external elastic lamina, cannot be visualised consistently by OCT, especially through lesions with a high amount of lipid-rich plaque burden. The relative merits of all the described intracoronary imaging modalities are shown in Table 2.
From a practical perspective one of the biggest differences between IVUS and OCT remains the need to replace the coronary blood pool with contrast during acquisition of OCT images. This involves the simultaneous injection of contrast to obtain the high definition images possible with OCT.47 The clinical value of the higher resolution images in guiding decision-making is still under evaluation.38
Studies Comparing Intravascular Ultrasound versus Optical Coherence Tomography
A recent prospective multicentre study (OCT Compared with IVUS in a Coronary Lesion Assessment [OPUS-CLASS] study) investigated the reliability of FD-OCT for coronary measurements compared with quantitative coronary angiography (QCA) and IVUS. Within a 100 patient cohort, both FD-OCT and IVUS exhibited good interobserver reproducibility, but the variability between measurements was approximately twice as high for the IVUS measurements as compared with the FD-OCT (0.32 versus 0.16 mm2).49 In addition, IVUS overestimated the lumen area and was less reproducible than FD-OCT (8.03 ± 0.58 mm2 versus 7.45 ± 0.17 mm2; p<0.001).49 FD-OCT therefore provided accurate and reproducible quantitative measurements of coronary dimensions in the clinical setting.
However, a recent randomised controlled trial comparing FD-OCT against IVUS for PCI optimisation reported that there was inferior stent expansion, both focal (65 versus 80 %, p=0.002) and diffuse (84 versus 99 %, p=0.003), when FD-OCT was used for guidance. PCI guided by FD-OCT also showed a significant increase in residual stent-edge plaque burden (51 versus 42 %, p<0.001). However, there were no significant differences in stent apposition.50 Therefore, this study found that IVUS had a significant advantage over OCT in terms of the reduction of residual stent-edge plaque burden and visibility of vessel border, which is in contrast to the results of the OPUS-CLASS study.49Table 3 is a summary of current clinical evidence for IVUS and OCT use.
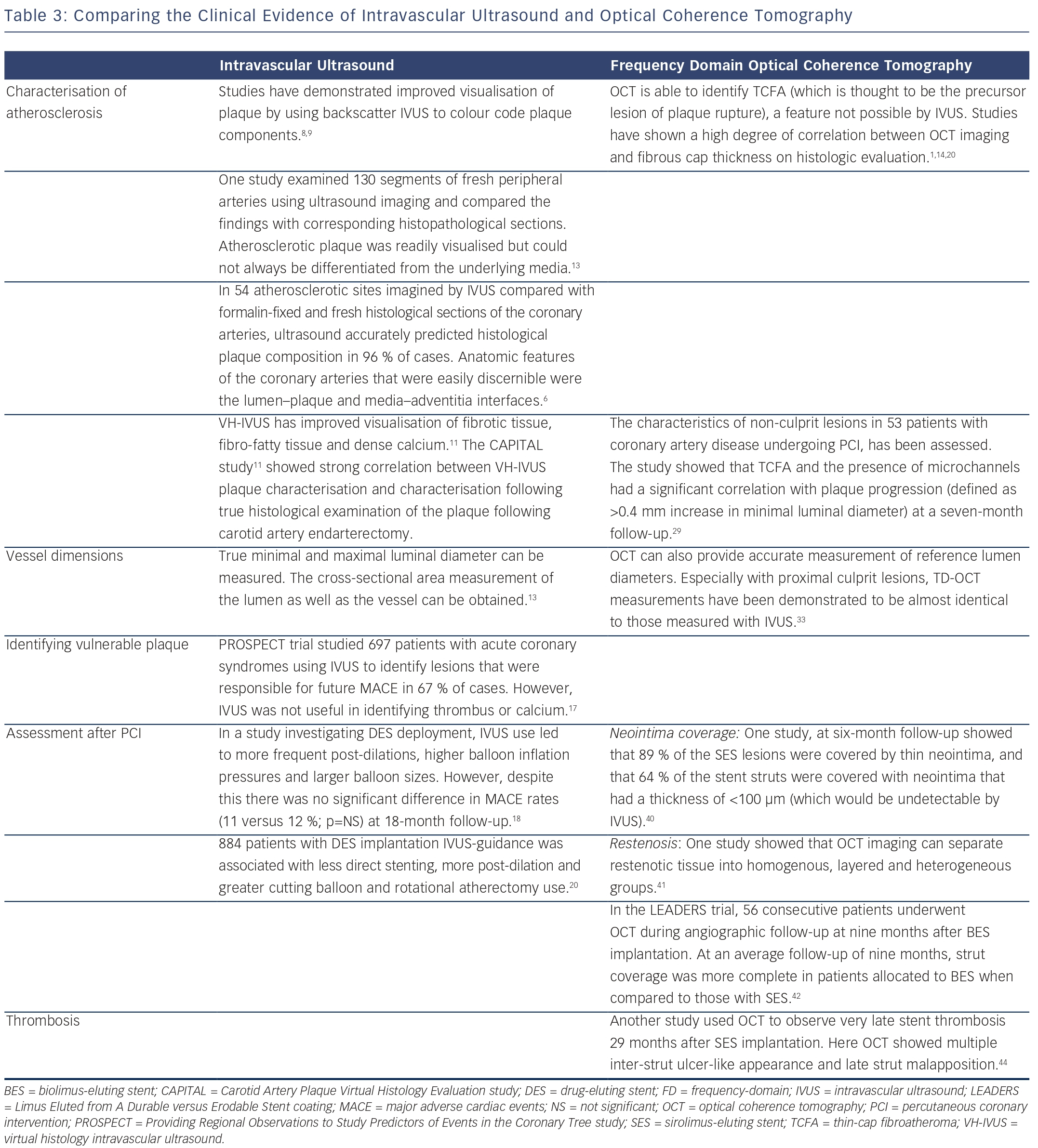
Current Clinical Practice Guidelines
The 2011 American College of Cardiology Foundation (ACCF)/American Heart Association (AHA)/Society for Cardiovascular Angiography and Interventions (SCAI) guidelines for PCI recommends the use of IVUS for the evaluation of angiographically indeterminate left main lesions and angiographically indeterminate (50–70 % stenosis) non-left main coronary lesions (Class IIa, Level of Evidence B recommendation).These guidelines also recommend the use of IVUS to evaluate the aetiology of stent restenosis and stent thrombosis (Class IIa, Level of Evidence C). The routine use of IVUS for evaluation of lesions when PCI is not planned was given a Class III recommendation.51
The 2010 European guidelines (European Society of Cardiology [ESC]) for Myocardial Revascularisation give a Class IIb, Level of Evidence C recommendation for the use of IVUS during unprotected left main PCI only.52 The lack of recommendation for other lesions or vessels appears to be related to limited data showing that IVUS reliably reduces MACE. However, the 2011 ACCF/AHA/SCAI guidelines do provide a Class IIa, Level of Evidence B recommendation for the use of IVUS for evaluation of donor coronary artery disease or allograft vasculopathy in post-cardiac transplantation patients.51
Currently neither the American (2011 ACCF/AHA/SCAI guidelines) nor European (ESC) guidelines provide recommendations for the routine use of OCT in clinical practice.51,52 However, more recent guidelines published in February 2014 by The National Institute for Health and Care Excellence (NICE)53 suggest that the evidence on the safety of OCT to guide PCI showed no major concerns. Due to the current available evidence on efficacy being limited in quantity and quality, it is recommended by NICE that this procedure should only be used with special arrangements for clinical governance, consent and audit or research.53
Future Clinical Research and Application of intra-coronary Imaging
OCT despite its extensive use in research studies has not yet been established in clinical practice and therefore currently should be seen as complementary to rather than replacing IVUS. However, it is expected that with the development of FD-OCT, the procedure will become both quicker and easier. As mentioned above, one major disadvantage of OCT is its limitation in the penetration depth (i.e. of approximately 2 mm). Therefore, although current OCT systems can demonstrate thin fibrous caps and thin neointimal coverage on DES, it is unable to quantify total plaque volume. Hence, development of new devices in conjunction with OCT might be helpful for both patient evaluation and clinical trials.
In addition, the need for optimal clearance of blood from the vessel lumen often requires extra doses of contrast to generate interpretable images. There are an increasing number of OCT studies being reported, which will hopefully further clarify the role of OCT in the near future. The FFR or OCT Guidance to RevasculariZe Intermediate Coronary Stenosis Using Angioplasty (FORZA) study will aim to compare the clinical and the economic impact of fractional flow reserve (FFR) versus OCT guidance in the percutaneous management of patients with angiographically intermediate coronary lesions.54 The DOCTORS study will evaluate the impact of changes in procedural strategy resulting from the use of OCT after angioplasty and stent implantation of a lesion responsible for NSTEACS.55
Finally, there has been little use of OCT in patients presenting with ST-elevation myocardial infarction (STEMI). Optical Coherence Tomography Assessment of Gender Diversity in Primary Angioplasty (OCTAVIA), is a recent study, which enrolled 140 STEMI patients who underwent primary PCI with an everolimus-eluting stent, and which demonstrated that at nine months, OCT showed that more than 90 % of patients had fully covered stent struts.56 Although this was a small study, it is likely that because of the superiority of OCT technology over IVUS, there will probably be many more studies that will use OCT to investigate plaque characterisation during primary PCI.
In addition to the rapid progress with OCT, future developments in IVUS are also expected with significant research ongoing indeveloping combinations of imaging modalities. Combining nearinfrared spectroscopy (NIRS) technology with IVUS allows better characterisation of lipid-rich plaque within a coronary artery.57 There are a number of ways by which NIRS-IVUS can help the optimisation of PCI and even play an important role in the prevention of spontaneous coronary events. Studies have suggested that NIRS has identified large, often circumferential lipid-rich plaques at the culprit site in most patients experiencing a STEMI.58 These data are now being translated into two large-scale prospective studies that will investigate the use of NIRS in the prediction of cardiac events beyond the success achieved with plaque burden in the PROSPECT Study.16,59
Conclusions
The development of OCT has markedly improved intracoronary image resolution compared with IVUS. OCT is superior to IVUS in a number of aspects, particularly distinguishing thrombus formation, coronary dissection and incomplete stent apposition following implantation. OCT also assists the characterisation of neointimal coverage after stent implantation and thrombus formation, thereby allowing early comparison of new technologies using intermediate endpoints. Both techniques are clearly useful in diagnosing, planning and evaluating the results of coronary intervention. Whether this provides a significant improvement to clinical decision-making is still debatable and intracoronary imaging therefore exists as a useful adjunct to clinical practice. The role in assessment of new technologies is more certain and the superiority of the imagesobtained using OCT is therefore more important. Whether OCT will replace IVUS as the clinical tool of choice for intracoronary imaging remains undetermined and will be guided by the results of ongoing clinical trials.