In the modern era, patients being treated with percutaneous coronary interventions (PCI) present an increasingly challenging profile in terms of both comorbidities and anatomical complexity. In this context, there is a growing awareness that performing ultra-low-dose contrast PCI contributes to increased safety and quality of the intervention.
Until recently, ultra-low contrast PCI, defined as a ratio of volume of contrast administered to estimated glomerular filtration rate (eGFR) <1, was envisaged as a one-indication strategy, namely for interventions in patients with chronic kidney failure at an increased risk of developing postprocedural contrast-induced nephropathy (CIN). From a broader perspective, ultra-low contrast PCI can be defined as an approach to PCI in which contrast administration is restricted, or even avoided completely, and in which procedural steps that customarily have been performed under angiographic guidance, such as guide catheter engagement, vessel wiring, location of target stenoses and optimisation of stent deployment, are performed using alternative contrast-free approaches.
CIN is a relatively common complication of PCI, accounting for approximately 11% of in-hospital acute kidney injuries, and is the third most common cause of renal insufficiency following impaired renal perfusion and nephrotoxic medications.1,2 Studies have also demonstrated that chronic kidney disease (CKD) is a frequently encountered comorbidity in patients with established coronary artery disease, with a prevalence of 23–46%.3–6 Definitions of ultra-low contrast PCI and CIN are provided in Table 1.
The approach to avoid CIN following PCI in patients with renal dysfunction has evolved significantly from a simplistic less-is-more PCI approach that, in many cases, was associated with inadequate stenting and incomplete revascularisation to a comprehensive suite of techniques encompassing dedicated skills and available physiology and imaging technologies, the aims of which are to increase the safety and quality of the intervention. For this reason, ultra-low contrast PCI has expanded its realm of applications to other interventional scenarios in which reducing the dependence of the operator on angiographic vessel opacification may contribute to better procedures.
This review highlights the latest practical and technological innovations that allow for the safe performance of ultra-low-dose contrast PCI in patients at risk of CIN and other complex scenarios, such that these techniques can be adopted into routine clinical practice.
Reducing the Dependence on Contrast During Percutaneous Coronary Intervention
In addition to the risk of CIN, there is jeopardy in performing low-quality PCI in complex cases where there are concerns over liberal contrast use, similar to the phenomenon called ‘renalism’ that has been described for coronary angiography.7 One of the key philosophies of the ultra-low contrast PCI techniques discussed herein is to prevent this new form of renalism. Where possible, the contrast-sparing techniques described below should be used not only to directly minimise renal injury, but also to enable sufficient contrast volume to complete high-quality procedures, especially given that a significant proportion of the patients at risk of CIN exhibit complex anatomy and can often require adjunctive intravascular imaging, physiology and plaque-modification techniques.
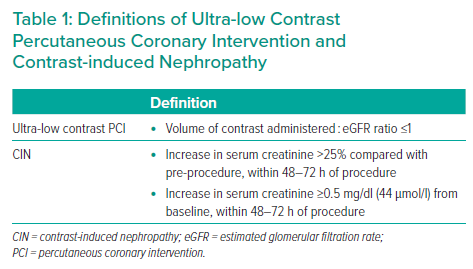
The optimum threshold of contrast volume:eGFR ratio is considered to be <1; hence, this is now used as the definition for ultra-low-dose contrast intervention.8–10 Although a number of definitions of CIN exist, the three key components include a relative or absolute increase in serum creatinine compared with baseline levels; a correlation between the rise in creatinine levels and the timing of contrast administration; and the exclusion of alternative explanations for the deterioration in renal function.2,11–13 The most frequently accepted definition of CIN is an absolute increase in creatinine of ≥0.5 mg/dl or a ≥25% increase from baseline 48–72 h after the administration of contrast.11 Although the overall risk of CIN in the general population is low, with a rate of 0.6–2.3%, it has been shown to occur in up to 20% of patients in high-risk subgroups.11 CIN has been associated with increased morbidity and mortality, longer hospital stays and poorer clinical outcomes.14,15
Typically, patients at risk of CIN have a number of predisposing risk factors that render them vulnerable to this complication.16 Eight independent variables have been identified as predictors of CIN: age >75 years, diabetes, chronic congestive heart failure, admission with acute pulmonary oedema, hypotension, anaemia and CKD.16 Several periprocedural risk scores exist, allowing the operator to objectively identify patients at greatest risk of developing post-PCI CIN.16–18
In addition to identifying patients at risk of CIN, preprocedural preparation is key for its prevention. Measures such as stopping nephrotoxic medications are strongly advised. In addition, correcting anaemia and hypotension is necessary. There is emerging evidence suggesting that targeted rather than blinded pre- and postprocedural hydration may be the optimal strategy because these patients exhibit high resting left ventricular end diastolic pressures (LVEDP).19 In the POSEIDAN trial, 396 patients were randomised 1 : 1 into two arms: LVEDP-guided volume expansion (n=196) or the standard fluid administration protocol (control group; n=200).19 In that study, contrast-induced acute kidney injury occurred less frequently in the LVEDP-guided group (6.7% [12/178]) than in the control group (16.3% [28/172]; RR 0.41, 95% CI [0.22–0.79]; p=0.005).19
Although there is a relative paucity of prospective randomised data investigating the combined use of ultra-low contrast techniques, the data suggest that this strategy can be deployed safely, with individual techniques extensively investigated.20 These techniques are discussed in conjunction with clinical scenarios below.
Clinical Scenarios for the Use of Ultra-low Contrast Percutaneous Coronary Intervention
There are a growing number of clinical scenarios in which ultra-low contrast PCI can be used. In addition to patients with underlying CKD, contrast- and volume-sparing techniques should be used where possible in patients with high-risk complex coronary disease (where reaching a high dose of administered contrast may be a limiting factor in continuing the procedure) and in shock (where renal hypoperfusion may exacerbate renal toxicity). Ultra-low contrast PCI techniques are also of great value when operating in iatrogenic or spontaneous coronary dissection to avoid injections that may cause hydraulic extension of the dissection. A summary of the most commonly encountered clinical scenarios in which to use ultra-low contrast PCI is provided in Table 2.
As discussed, patients with CKD are at risk of acute renal deterioration in the presence of high volumes of iodinated contrast. Controlled and sparing use of contrast is paramount in maintaining stable renal function. The degree of CIN can also be predicted to a certain degree, and patients at risk are often diabetic and/or undergoing treatment with multiple nephrotoxic pharmacotherapies. In these patients, although nephrotoxic medicines can be temporarily halted prior to the intervention and preprocedural hydration initiated, ultra-low contrast techniques should still be used.
Cardiogenic shock is associated with poor renal outcomes, and patients who undergo percutaneous revascularisation are at greater risk of CIN.21,22 Although the precise molecular pathways that lead to renal dysfunction are incompletely understood, it is likely that the mechanisms of CIN in cardiogenic shock and other syndromes that precipitate acidaemia and/or hypovolaemia are overlapping. Preprocedural renal volume depletion, acidaemia, the presence of reactive oxygen species and depletion of nitric oxide are all likely key precipitants of renal medullary vasoconstriction.23 Osmotic changes in interstitial pressure and sodium–potassium transporter stimulation can cause cellular oedema and hypoxia and worsen the cycle of CIN.24 Therefore, shock is a significant risk factor for CIN, and in these cases, ultra-low contrast administration has the benefit of reducing these maladaptive cellular changes.
In coronary artery dissection, contrast administration should be avoided to prevent further hydraulic extension of the dissection flap. In spontaneous coronary artery dissection, contrast administration should be limited with selective projections and short contrast runs. In instances of iatrogenic coronary dissection, the vessel will likely require stenting; however, the techniques described below will offer guidance to prevent extension of the dissection flap and gain control of the clinical situation through successful PCI of the true lumen.
In complex high-risk indicated PCI and chronic total occlusions, excessive contrast load can precipitate CIN. Complex high-risk patients undergoing PCI are at risk of impaired haemodynamics and persistently low blood pressure through impaired left ventricular function or fixed aortic valve outflow obstruction. Given that this specific group of patients occasionally requires mechanical circulatory support, ultra-low contrast techniques should be used for reasons similar to those for patients in shock. In addition, in prolonged and complex procedures, contrast sparing prevents the need to interrupt or stage a procedure and allows for more room in completing the entirety of the planned intervention.
There are several key challenges encountered by the operator when performing ultra-low contrast PCI. Although the key aim of the ultra-low contrast technique is to limit, where possible, the liberal usage of contrast, in almost all cases the use of contrast cannot be eradicated completely. The guidance described herein aims to reduce the dependence on contrast for confirmation of guide catheter engagement, wire navigation, target lesion identification, adequate balloon dilatation and deployment, assessment of the results of the procedure and, importantly, the identification and management of complications. These techniques are described in greater detail below.
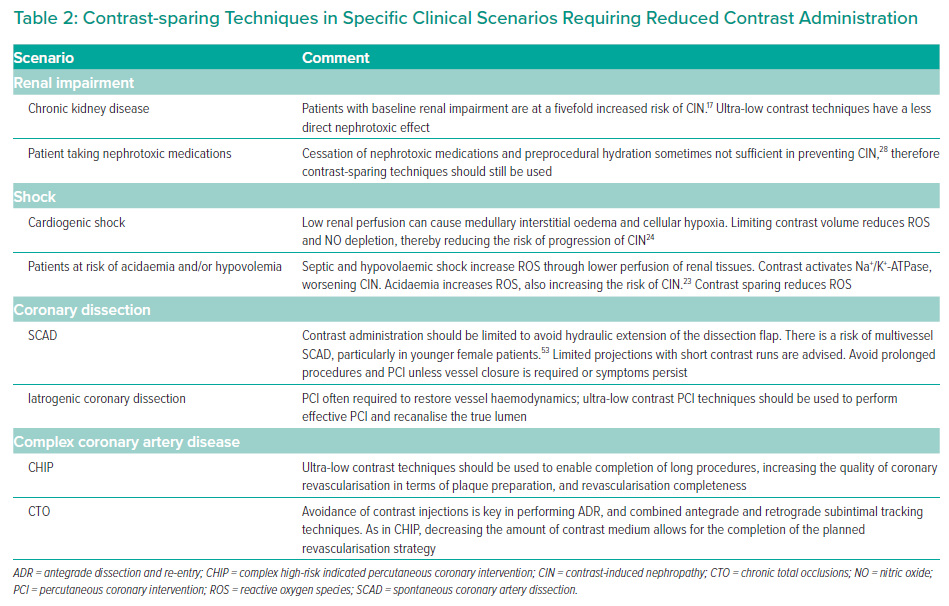
Techniques for Reducing Contrast Volume Administration
Guide Catheter Engagement
In the context of ultra-low-dose contrast angiography, it is prudent to choose smaller catheters (5–6 Fr) without side holes, to ensure maximum opacification of the vessels with each contrast injection. There are a number of useful strategies that can be used to reduce the requirement for contrast in confirming catheter engagement. Coronary calcium can be used as a guide to locate the coronary artery ostia and silhouette, and is frequently observed in patients with CKD.25 By increasing the frame rate, calcium can be more readily identified.26 Calcium can also be used as a reference landmark within the vessel to guide stent placement in PCI.
In the study by Kim et al., either 0.9% saline or 5% dextrose solutions were injected into the left coronary artery before measuring heart rate, the QT interval and T wave amplitude.27 The authors reported T wave amplitude changes in a significant number of patients injected with 0.9% saline.27 By injecting 10–20 ml of 0.9% saline through the catheter, correct positioning of the catheter can be confirmed, with T wave inversion or increased amplitude, in addition to ST-segment depression or elevation.26,27 An example of this is shown in Figure 1.
When performing a diagnostic angiogram, it is essential to minimise the number of projections. To visualise the left coronary artery, using the anteroposterior cranial and caudal projections allows for optimal visualisation. The left anterior oblique with cranial projection is optimal for visualising the right coronary artery. It is important to avoid panning and to use low magnification because these angiograms can be used as a road map if PCI is required. Using diluted contrast (a 1 : 1 or 1 : 2 ratio of saline to contrast) allows more contrast injection runs, albeit at the expense of luminal opacification.28 Using higher frame rates may aid the operator to maximise visualisation of the coronary anatomy with each injection.26 This is of particular importance if using diluted contrast. Where doubts exist regarding the significance of a stenosis seen on angiography, the use of other techniques (e.g. coronary physiology) or intracoronary imaging must be considered rather than additional angiographic views to determine whether the given lesion requires intervention. If PCI is required, this should be performed as a staged procedure with ideally 1 week between the two procedures.9
Catheter Flushing
Some experienced operators in ultra-low-dose contrast interventions choose to remove excess contrast from the guiding catheters following contrast administration. This is particularly relevant prior to catheter exchange or drug administration in order to prevent excess contrast being administered unnecessarily.26 It has also been reported that it is feasible to remove the excess contrast by aspirating the coronary sinus immediately following contrast injection. A small study of 43 patients with type 2 diabetes and CKD who were undergoing PCI compared standard of care to coronary sinus aspiration with an 8.5-Fr transeptal sheath, which resulted in the removal of more than one-third of the contrast.29 The CIN rate was lower in the coronary sinus aspiration group than in the control group (5.5% versus 36%; p=0.03). However, eGFR and the contrast volume to creatinine clearance were not reported, limiting the interpretation of the efficacy of this technique.28,29
The effectiveness of the use of the DyeVert PLUS system (Osprey Medical) has also been explored.30,31 This system is connected between the injection syringe and manifold and diverts the excess contrast into a reservoir chamber.28 Mehran et al. conducted a study of 587 high-risk patients and demonstrated that the use of this system was associated with a 15.5% relative reduction in contrast volume (mean [±SD] 85.6 ± 50.5 versus 101.3 ± 71.1 ml; p=0.02), however this did not translate into a reduction of CIN.30 In a second smaller study using a newer version of the DyeVert system, a 40% reduction of contrast volume was observed.28,31
Wire Navigation
When the coronary anatomy is already known, diagnostic cine images can be displayed in real time during the PCI and used as a road map to engage the catheter and treat the vessel in question.26 This can be performed with relative ease by displaying the diagnostic images in real time on a separate monitor during the procedure, with the operator using the same projections for the PCI. Using the diagnostic images as a reference, multiple guidewires can be used to recreate the coronary anatomy by wiring the main branch and relevant side branches, taking care to ensure that the guidewires follow the exact course of the vessel seen on the diagnostic images, as shown in Figure 2.9 By ensuring guidewires without hydrophilic coatings are used, and by shaping the workhorse wire to create a knuckle once it has crossed the stenotic lesion, the risk of distal perforation can be reduced.26 By creating a metallic outline of the vessel and its side branches and combining these with the diagnostic images, a better understanding of the anatomy can be gained, reducing the need for contrast injection or puffing. Experienced operators may elect to use larger, more supportive 7-Fr guiding catheters in the case of complex anatomy to facilitate the use of multiple coronary wires, intravascular ultrasound (IVUS) catheters and guide extension catheters, which can be advanced via radial access in most cases.9,26
The marking wire technique has also been described, which aids in the determination of the proximal and distal landing zones in ultra-low-dose contrast PCI.32 This technique involves the insertion of two guidewires, via a double Y connector, and a 7-Fr guide catheter. The main operating wire is inserted via the main port of the connector and a second wire, the marking wire, is inserted via the side port. IVUS should then be performed to determine the distal landing zone; the marking wire is then pulled back to the level of the landing zone. By closing the connector’s side port and applying a torquer, the risk of the marking wire moving is low. Lesion length and the proximal landing zone are then determined by IVUS. Subsequently, the stent is advanced to the distal landing zone, as marked by the wire, and, at this point, the marking wire may be removed. Following implantation, IVUS should be performed to ensure optimal results and confirm the absence of complications and geographical miss.32 Using these techniques and creating roadmaps with coronary wires will aid the operator in creating a representation of the coronary anatomy, reducing the need for repeated contrast injections.
Dynamic Coronary Roadmapping
The Dynamic Coronary Roadmap (DCR; Philips Medical Systems Nederland BV) is software that offers a real-time dynamic overlay of the coronary tree on fluoroscopy. The roadmap is generated automatically following a single angiogram and can be used as a navigational tool during PCI. This software allows the operator to store and easily re-display previously acquired roadmaps, which may enhance procedure efficiency. In ultra-low-dose contrast PCI, this technology is useful and can be used not only for the wiring of main vessels and side branches but also for the advancement and precise positioning of balloons and stents (Figures 1 and 3), reducing the need for contrast administration.33
In a feasibility study by Piayda et al., 936 overlay runs created during 36 PCI procedures were analysed.33 That study assessed both roadmap quality (correct dynamic imaging of the vessels without relevant artefacts or missing parts) and overlay quality (congruence of dynamic coronary roadmapping and coronary anatomy). In addition, the authors assessed procedural success and the occurrence of major adverse cardiovascular events (MACE). Roadmap quality was defined as adequate and safe for clinical use in 99.5% of vessel analyses, with overlay quality being sufficiently good in 97.4%.33 Overall, low inter- and intra-observer variability were observed (intraclass correlation coefficient R=0.84 for roadmap quality and R=0.75 for overlay quality). The procedural success rate was 100%. MACE occurred in two (5.6%) patients during the postinterventional in-hospital stay and was not software related in either case.33 In a study by Yabe et al., 150 patients were included (43 treated with the DCR system and 107 in the normal treatment group).34 That study demonstrated significantly lower fluoroscopy time (mean ± SD: 11.4 ± 5.5 versus 17.5 ± 13.4 min; p<0.01) and contrast volume (118.8 ± 49.7 versus 158.7 ± 86.9 ml; p<0.01) in the DCR than normal treatment group.
The results of these small-scale studies are encouraging, but larger studies are warranted to assess whether the technology reduces both contrast medium administration and radiation doses. The ongoing randomised and multicentre Dynamic Coronary Roadmap for Contrast Reduction Trial (DCR4Contrast; NCT04085614) will attempt to address these questions and further validate the technology.
Intracoronary Imaging and Physiology
In order to perform ultra-low-dose and zero contrast PCI, rather than relying on traditional coronary angiography, we can also depend on intracoronary imaging and invasive coronary physiology to perform the PCI safely and optimise the results. Operators in the modern era should be incorporating these techniques in their daily practice regardless, and the need for the use of coronary physiology and IVUS is magnified in this context. Studies have shown that the use of IVUS and invasive coronary physiology is associated with lower rates of MACE and improved clinical outcomes.35–40 Not only should these techniques be routinely used to determine whether interventions are indicated, but they should also play a pivotal role in the assessment of plaque characteristics, lesion length and vessel sizing, as well as in the evaluation and optimisation of post-PCI results. This has been reflected in the most recent myocardial revascularisation guidelines.41
IVUS has traditionally been the intracoronary imaging method of choice in this clinical context. In the MOZART trial, 83 patients were randomised to angiographic-guided PCI or IVUS-guided PCI.42 The primary end point for this trial was total volume of contrast administered during PCI. In this trial, operators were encouraged to use contrast-sparing methods in both arms. The median total volume of contrast was 64.5 ml (interquartile range [IQR] 42.8–97.0 ml; range 19–170 ml) in the angiography-guided group, compared with 20.0 ml (IQR 12.5–30.0 ml; range 3–54 ml) in the IVUS-guided group (p<0.001).42 In that study, most patients had normal renal function at baseline, and the study was not powered to detect a difference in post-PCI renal function. Both study arms primarily comprised diabetic patients with long and complex lesions. It should also be noted that the angiography-guided PCI arm also had a low volume of contrast administered, which could be due to strict contrast-saving strategies used in the protocol. Although a small study, the findings support the use of IVUS in these clinical scenarios, and highlight its safety while reducing the volume of contrast administered threefold.42 The ongoing MOZART II study (NCT02743156) aims to recruit 300 patients and assess whether the use of IVUS may lead to a decrease in the risk of CIN after PCI within 72 h of the procedure.
In a feasibility study by Ali et al., 31 patients with advanced CKD (median creatinine 4.2 mg/dl [IQR 3.1–4.8 mg/dl], mean [±SD] eGFR 16 ± 8 ml/min/1.73 m2) underwent PCI without contrast.9 All patients had previously undergone a diagnostic coronary angiogram with ultra-low-dose contrast administration at least 7 days prior to the PCI, with these images used as references during PCI. Each patient had an echocardiogram prior to the procedure to out rule a pericardial effusion, and targeted intravenous hydration was performed based on left ventricular end-diastolic volumes. The operators then confirmed guide catheter placement by advancing a workhorse wire into the coronary artery. A metallic silhouette of the coronary anatomy was created and used as a roadmap to mark important landmarks. Fractional flow reserve and coronary flow reserve were recorded before and after the PCI. Procedural planning was performed using IVUS guidance to identify proximal and distal landing zones, for vessel sizing and determining lesion length. A ‘dry cine’ using the coronary wire allowed for the co-registration of images. Following PCI, IVUS was repeated to evaluate and optimise the PCI result, and finally, the invasive physiology was repeated to ensure an optimal physiological result. At a median follow-up of 79 days (IQR 33–207 days), neither creatinine levels (median 3.7 mg/dl [IQR 3.0–4.5 mg/dl]; p=0.69) nor eGFR (median 18 ml/min/1.73 m2 [IQR 14–22 ml/min/1.73 m2]; p=0.70) were significantly different, and no patient required renal replacement therapy (RRT). There was no increase in MACE at the short-term follow-up in all patients.9 Similarly, a study of 20 patients by Sacha et al. demonstrated that IVUS-guided zero contrast PCI in patients with advanced CKD was feasible and was associated with good clinical outcomes at a median follow up of 3.2 months (IQR 1.2–5.3 months).43
Sakai et al. assessed the clinical impact of IVUS-guided ultra-low-dose contrast PCI in patients with stage 4 or 5 CKD.44 The primary end point was all-cause mortality, MI and the induction of RRT at 1 year following PCI. Compared with the angiographic-guided group, there was a significant reduction in contrast volume administration (mean ± SD: 22 ± 20 versus 130 ±105 ml; p<0.0001) and CIN (2% versus 15%, p=0.001) in the IVUS-guided group. Success rates were similar in both groups. At 1 year, there was a lower rate of RRT in the IVUS-guided group (2.7% versus 13.6%; p=0.01); however, all-cause mortality and MI were similar in the two groups.44 Although these are small-scale studies, they are thought provoking, and help demonstrate the utility of IVUS and coronary physiology in this emerging field, in addition to previously mentioned contrast-reducing techniques.
Co-registration of Intracoronary Imaging and Physiology with the Coronary Angiogram
Significant advances have been made in the co-registration of physiology and IVUS with coronary angiography. When ultra-low-dose contrast intervention is required, the physiology and IVUS may be co-registered using the coronary wire as a surrogate for angiography. The SyncVision Precision Guidance System (Philips Volcano) is currently commercially available. It allows the operator to perform a physiological assessment with an instantaneous wave-free ratio (iFR) and co-register it with the angiographic image, allowing for the measurement of lesion length and prediction of the physiological outcome of treating a given segment, as shown in Figures 1 and 3. By displaying yellow dots, representing a 0.01-iFR unit of step up on the co-registered angiogram, it provides the operator with a visual display of the physiological significance of angiographic lesions. Kikuta et al. evaluated the accuracy of the iFR pull-back measurements to predict post-PCI physiological outcomes and how frequently the iFR pull-back changed the PCI strategy.45 The study enrolled 128 patients with paired pre- and post-PCI iFR measured in 134 vessels. The operators were asked to submit a procedural plan once angiography had been performed, and then again after the iFR pull-back incorporating virtual PCI with a predicted final iFR result. A repeat iFR assessment was performed after the PCI had been performed. The mean (±SD) predicted post-PCI iFR calculated online was 0.93 ± 0.05; the observed actual iFR was 0.92 ± 0.06. The iFR pull-back predicted the post-PCI iFR outcome with an error of 1.4 ± 0.5%, and altered the decision-making process in 31% of vessels.45
The SyncVision IVUS co-registration feature affords the operator an opportunity to combine the IVUS and angiographic appearance of the vessel, providing co-registration of the IVUS images on the angiogram and facilitating measurements of lesion length and vessel size, as well as assessment of plaque characteristics. A pilot study by Frimerman et al. assessed data from 42 arteries in 36 patients following an initial validation using an offline phantom model study.46 The phantom study reported a co-registration length accuracy of 1.12 mm. The operators used a grading system from 1 to 5 to evaluate the following: accuracy (mean ± SD: 4.8 ± 0.4), ease of use and workflow (4.7 ± 0.4), stent landing zone detection and evaluation (4.6 ± 0.5), stent landing zone length, and diameter measurement (4.9 ± 0.2).46 This system automatically integrates the IVUS pull-back run with the angiography through video acquisition, and takes less than 30 s to complete. Once completed, the operator can move a marker along the angiographic image displayed on one screen and view the corresponding IVUS images of the same location.46 The SyncVision system also allows for the iFR and IVUS to be tri-registered, combining all the above features, and is, therefore, a more comprehensive evaluation. In addition, this system offers the SyncVision Angio+ device inflation and enhancement technology, which allows for enhanced visualisation of balloon and stent deployment under fluoroscopy to ensure accurate localisation and deployment, and the avoidance of geographical miss.47
A key aspect of the use of the tools described above in the context of ultra-low contrast PCI is performing co-registration not with the angiographic image of the vessel, but with the intracoronary guidewire instead. With the obtained information, the operator may locate flow-limiting disease, select the best available landing zones for stenting, decide whether plaque preparation is needed and optimise implanted stents; all can be achieved without the need for delivering contrast medium to the patient (for examples of wire-based co-registration, see Figures 2and4).
There are limited data on the use of optical coherence tomography (OCT) without the use of contrast using saline and colloid infusate, or dextran, to displace blood and to guide PCI.10,28,48–50 In a study of 51 vessels in 26 patients, using dextran in place of contrast resulted in similar image quality and measurements.49 Azzalini et al. also reported that it is feasible to use dextran as a substitute for contrast, although it must be kept in mind that this agent is not free of nephrotoxic effects.10 Some authors have reported using guide extension catheters to perform more selective injections of saline, thus achieving more effective removal of blood over OCT image acquisition.51,52 Overall, however, there is a paucity of data in this area, and further studies are required.
It should be noted that there are perceived limitations to performing ultra-low and/or zero-contrast PCI, particularly with regard to suboptimal stenting results due to reduced angiographic visualisation. Ultra-low contrast PCI procedures should only be embarked upon if intracoronary imaging and physiology are used to ensure precision and optimal PCI results. Although intracoronary imaging techniques typically detect complications, such as dissections, the use of a final contrast run of the treated vessel is encouraged to ensure visualisation of potential complications, such as distal wire perforations or side branch occlusions.
A summary of the practical components to perform ultra-low contrast PCI is shown in Figure 5.
Conclusion
There is growing awareness of ultra-low contrast PCI, and the need for these interventions is likely to increase given the increasing anatomical complexity of patients encountered in the cath lab. There is an increasing body of evidence supporting that ultra-low contrast PCI can be performed safely. These interventions require a mindset change and challenge operators to upskill and to become accustomed to incorporating contrast-saving techniques in their daily practice.
Simple changes, such as displaying diagnostic images during PCI cases or using additional coronary wires to mark pertinent anatomy, can be useful. Intracoronary imaging and invasive physiology should be used, where possible, in place of contrast to ensure optimal PCI results. The evolution and wider availability of navigational technology, such as dynamic coronary roadmapping and co-registration technology, have refined and streamlined these interventions.
It is likely that with ongoing development of the technologies described and continuous innovation, in conjunction with awaited prospective randomised clinical studies, ultra-low-dose contrast interventions will become more common in cardiac cath labs and will improve the breadth and effectiveness of percutaneous revascularisation techniques in patients with renal dysfunction, as well as those undergoing prolonged complex procedures.